Congratulations, you just discovered the best article in the planet! Make sure you follow through till the end ok?
Thanks for reading this post, don't forget to subscribe!
Tech that Mimics Life: The Future of Biocomputing
Last updated: April 23, 2025
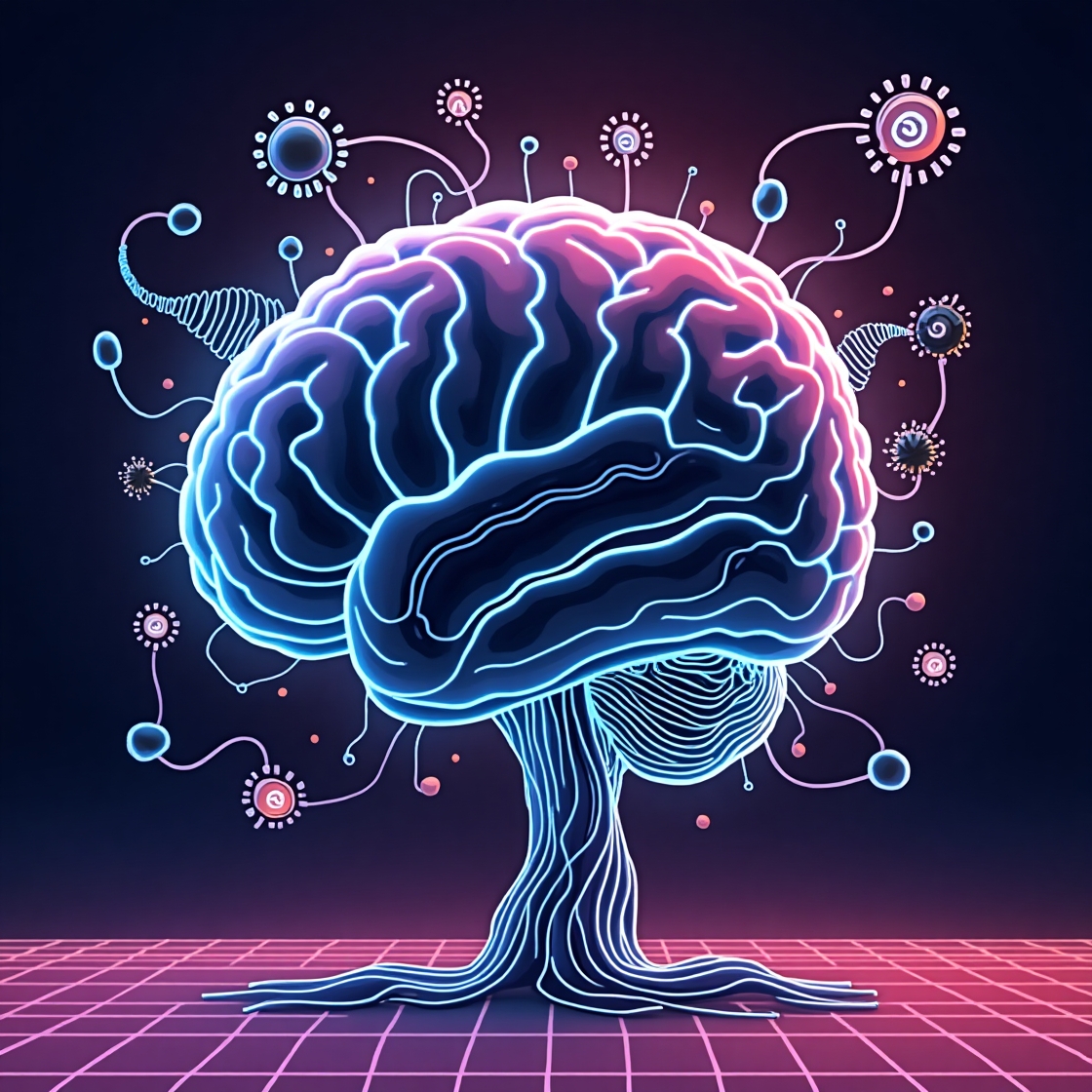
Introduction
Biocomputing represents the convergence of biology, computer science, and engineering to create systems that process information using biological molecules or bio-inspired architectures. As we move into 2025 and beyond, biocomputing promises to revolutionize data storage, medical diagnostics, and artificial intelligence by leveraging mechanisms found in living organisms.
What Is Biocomputing?
Biocomputing encompasses:
- DNA Computing: Using DNA strands for information encoding and parallel computation.
- Protein-Based Logic Gates: Engineering proteins to perform logical operations.
- Neuromorphic Systems: Hardware that mimics neural networks and brain architecture.
- Synthetic Biology Circuits: Designing gene circuits for programmable cellular behavior.
Key Biocomputing Technologies
1. DNA Data Storage
DNA offers ultra-high density storage—one gram can store over 215 petabytes. Recent advances have improved read/write speeds and error correction.
2. DNA Strand Displacement Circuits
These circuits use programmable DNA interactions to perform computational tasks like signal amplification and pattern recognition.
3. Protein-Based Computing
Engineered proteins serve as switches and logic gates, enabling computation within cellular environments.
4. Neuromorphic Chips
Chips like Intel’s Loihi mimic neuronal spiking behavior, offering energy-efficient AI processing.
5. Gene Circuit Engineering
Synthetic gene networks program cells to sense and respond to environmental signals, with applications in biosensing and therapeutics.
Applications Across Industries
- Healthcare: Rapid diagnostics, personalized medicine, and smart drug delivery systems.
- Data Storage: Archival of vast datasets in DNA for long-term preservation.
- Environmental Monitoring: Engineered organisms detecting toxins or pollutants.
- Artificial Intelligence: Bio-inspired hardware for low-power, high-efficiency AI.
Technical and Ethical Challenges
- Scalability: Manufacturing molecular components at scale.
- Error Rates: Reducing biochemical reaction errors in DNA computing.
- Ethical Concerns: Biosafety, dual-use research, and intellectual property.
- Regulatory Frameworks: Establishing standards for clinical and commercial applications.
Future Trends & Innovations
Emerging directions include:
- Hybrid Bio-Electronic Systems: Seamlessly integrating biological components with silicon circuitry.
- In Vivo Biocomputing: Implantable biological sensors performing computation within the body.
- AI-Guided Biodesign: Using machine learning to optimize genetic circuits and molecular devices.
Recommendations for Researchers
- Pursue interdisciplinary collaborations across biology, engineering, and computer science.
- Invest in standardized protocols for molecular manufacturing and data encoding.
- Engage ethicists early to address societal impacts and biosafety.
- Publish open-access datasets and design files to accelerate innovation.
Frequently Asked Questions
1. How does DNA computing differ from traditional computing?
DNA computing uses biochemical reactions for parallel processing, offering massive concurrency but slower individual operations compared to electronic computers.
2. What are the storage lifespans of DNA data archives?
Properly stored, DNA can preserve data for centuries to millennia, far surpassing magnetic or optical media.
3. Are biocomputing systems safe for medical use?
Clinical applications require rigorous biosafety testing. Synthetic circuits must include fail-safes and kill-switches to prevent uncontrolled behavior.
4. Can protein-based logic gates operate in living cells?
Yes, engineered proteins have been demonstrated to perform logic operations in vitro and in simple cellular systems, with ongoing research to improve reliability.
5. What is the largest barrier to commercializing biocomputing?
Scalable production and integration with existing electronic infrastructure remain significant hurdles, alongside regulatory approval processes.
References
- Adleman, L.M. (1994). “Molecular Computation of Solutions to Combinatorial Problems.” Science.
- Church, G.M. et al. (2012). “Next-Generation Digital Information Storage in DNA.” Science.
- Silver, P.A. & Way, J.C. (2017). “Engineering Biology.” Cell.
- Indiveri, G. et al. (2011). “Neuromorphic Silicon Neuron Circuits.” Frontiers in Neuroscience.